Michael Kogan, MD, PhD Member, International Neuromodulation Society University of New Mexico School of Medicine, Department of Neurosurgery Albuquerque, New Mexico, U.S.A.
Chengyuan Wu, MD, MSBmE Member, International Neuromodulation Society Thomas Jefferson University Hospital, Department of Neurosurgery Philadelphia, Pennsylvania, U.S.A.
Epilepsy is characterized by recurrent seizures and is the third most common neurological disorder in the world, with 0.5 - 1% of the population suffering from the disease. (1) In 20 - 40% of these patients, medications alone are unable to adequately control seizures and are therefore diagnosed with drug-resistant epilepsy, referred to as medically refractory. (1) For 60% of patients with drug-resistant epilepsy, surgery can significantly reduce or eliminate seizures. (2, 3) Despite such treatments, 15 - 40% of all patients with epilepsy unfortunately continue to have seizures that impair their daily living. (4) In an effort to improve the quality of life for these remaining patients, both epileptologists and neurosurgeons have been turning to the use of neuromodulation – the therapeutic application of electric currents to the nervous system. (5)
Development of Brain Stimulation for Neurological Disorders
In the 1940s, the observation that damage to the basal ganglia structures in the brain could lead to improvement in Parkinson disease’s symptoms lead to the use of surgical lesioning for the treatment of the disease. (5) Over time, a reversable form of this therapy through electrical stimulation was developed as an alternative to permanent lesioning. (6) By 1996, the FDA approved deep brain stimulation (DBS) for treatment of motor symptoms in appropriate patients with movement disorder. This application was later expanded to include other conditions, such as dystonia in 2002.
Types of Neuromodulation Used for Medication-Resistant Epilepsy
The idea of neurostimulation for medication-resistant epilepsy also started to take root near the time that neuromodulation was being developed for movement disorder. In the decades since, the therapy has grown dramatically. Neuromodulation for epilepsy began with the concept of vagus nerve stimulation (VNS), which received FDA approval for epilepsy in 1997 for adults and adolescents with partial onset seizures; the approval was extended to children as young as 4 years of age in 2017. (7, 20) Meanwhile, the responsive neurostimulation system (RNS) received FDA approval in 2014. In 2018, the FDA approved epilepsy with DBS that targets a brain structure called the anterior nucleus of the thalamus.
These neuromodulation approaches for epilepsy, VNS, RNS, and DBS, will be described below, beginning with the first neuromodulation treatment for epilepsy, vagus nerve stimulation.
Vagus Nerve Stimulation (VNS)
A VNS neuromodulation device delivers neurostimulation pulses through a thin, flexible electrical lead that is attached to an implantable pulse generator. The clinician programs the stimulation to be delivered, and the patient is sent home with a small hand-held controller that operates within those parameters. During implantation, a flexible electrical lead is tunneled under the skin of the neck. The end of the lead is tipped by electrical contacts, which encircle the vagus nerve that runs along the side of the neck. To power the system, the lead is attached to a pulse generator that is implanted under the skin in the upper chest wall.
Selection of Epilepsy Patients for Vagus Nerve Stimulation (VNS)
Any refractory epilepsy patient may be considered a candidate for VNS. While there are beneficial results for some patients, this option does not quite approach the efficacy of deep brain stimulation. However, VNS does present an opportunity to treat refractory seizures without the need for an intracranial procedure.
In the largest cohort study of 195 patients with refractory epilepsy, 35% of patients reported 50% reduction of symptoms at 12 months. (7) Furthermore, 20% experienced a 75% reduction in seizures. Interestingly, VNS has also been shown to improve mood. In a randomized control study, patients who received high-amplitude stimulation had statistically improved depression and anxiety measures at 3 and 6 months. (17)
Responsive Neurostimulation (RNS) for Medication-Resistant Epilepsy
A closed-loop RNS system only delivers stimulation when it detects the beginnings of seizure activity. In that way, it functions much like a pacemaker does to stop abnormal heart rhythms. Such stimulation works to either reduce the risk of having a seizure altogether or stops seizures from spreading to other parts of the brain.
Patients who are evaluated for traditional epilepsy surgery often need to undergo an initial surgical procedure to determine exactly where the seizures start. To do this, patients are monitored for seizures during a hospital stay. Brainwaves are monitored using an electroencephalogram (EEG), which involves placing electrodes on the brain surface, with wires exiting out through the skull and scalp. Once a seizure occurs, doctors can use the EEG to pinpoint the area of the brain that is causing seizures and define the target for surgery.
After promising results were observed in animal testing, closed-loop stimulation was studied in patient volunteers who were undergoing this type of invasive monitoring. (23) At first, a doctor who saw the beginnings of seizure activity on the EEG would initiate a pulse of stimulations. It was seen that in response to that method, abnormal EEG activity could be effectively stopped. (41-62) In order for a device to function independently, however, reliable seizure detection methods had to be created. In closed-loop stimulation, the first half of the loop is seizure detection, and the second half of the loop is the resulting stimulation. Over the past few decades, seizure detection has matured significantly. Scientists and engineers have developed sophisticated signal processing to more reliably detect the onset of seizures. (27-36)
Studies in the early 2000s showed that closed-loop stimulation provided patients therapeutic benefit with no major side effects. Direct stimulation of the region of the brain suspected to start the seizures (the seizure onset zone) was found to be at least as effective in stopping seizures as indirect stimulation with DBS. (37) Patients not only had fewer seizures, but their remaining seizures were also less severe. (1, 37, 38)
Since a closed-loop system provides stimulation only when triggered by early seizure activity, the brain receives less stimulation than it would with earlier types of neuromodulation, which deliver constant stimulation. This reduction in stimulation dose not only helps the battery last longer, but also decreases the potential risk of side effects from long-term continuous stimulation. (41-43) In fact, it has been shown that twice as much stimulation can be safely given with intermittent systems when compared to constant stimulation systems. (42)
Another benefit of closed-loop systems is that stimulation is targeted only at the seizure focus. In all other forms of neuromodulation (VNS, TNS, and DBS), stimulation is applied to seizure-generating circuitry and affects many parts of the brain as well. By isolating the seizure focus, normal parts of the brain are left alone and the chance of side effects is further reduced. (58)
Expected Outcomes with Closed-Loop Stimulation
These early studies laid the foundation for a large clinical trial carried out between 2005 - 2007 across multiple hospitals in the United States. The RNS System Pivotal Clinical Investigation included 191 adult patients with medically refractory epilepsy who were implanted with a closed-loop system at 31 institutions. (39, 41) Patients in the study group had their system turned on for the first 12 weeks, while those in the sham group, to which the study subjects were compared, received no stimulation. During this initial 12-week period, it was noted that patients with an active system averaged 41.5% fewer seizures. At the same time, the sham group only had a 9.4% reduction in the frequency of their seizures. (40) All patients had their system turned on after the initial 12 weeks to see the long-term effects of closed-loop stimulation. After two years, almost half of the participants had at least a 50% reduction in seizure frequency. (39, 41)
Longer-term follow-up shows an increase in the percentage of patients responding to RNS stimulation over time. (39) A 2011 update on these 191 implanted patients gave strong evidence that closed-loop stimulation significantly reduced the frequency of disabling seizures in patients who had previously tried not only anti-seizure medications, but also VNS or traditional epilepsy surgery. (40) With long-term follow-up of an average of six years, patients benefited from approximately a 70% decrease in their seizures. Furthermore, 29% benefited from a seizure-free period of at least six months; with this extending to at least one year in 15% of patients. (60) More important than reductions in seizure frequencies are the significant improvements in quality of life reported by these patients. (40, 45) Long-term follow-up has also shown that there are no negative effects of chronic stimulation on mood or cognition. (47, 48)
The RNS Device
The first closed-loop stimulation system to be implanted is the responsive neurostimulator system (RNS) by NeuroPace, Inc. (Mountain View, CA). (48) It consists of a main component, about the size of a pocket watch (measuring 4 x 6 x 0.7cm) connected to two electrodes. (38) The main component contains a battery and a computer that can analyze EEG readings and administer stimulation once it detects a seizure onset. This device can also store small snippets of EEG data, which can be downloaded to a NeuroPace computer, which in turn, can transfer the data securely to a central server where the patient’s neurologist can see the data.
Two types of electrodes can be used in any combination with this system. One type (a subdural electrode) consists of a strip of four disc-shaped contacts that is placed on the surface of the brain. The other type (a depth electrode) is made up of a strip of cylindrical contacts that is placed into the brain tissue itself. Both types of electrodes are made of a combination of platinum and iridium, which is able to safely administer therapeutic stimulation without generating a harmful reaction from the surrounding tissue. (38, 54)
Components of the system that are not implanted include a physician programmer, a patient data transmitter used for storing EEG data, and a telemetry wand for wireless transmission of data. (42) These tools allow doctors to transfer the recorded information to a computer for detailed analysis. By doing this, the clinician can see how the device is working, and fine-tune the stimulation programs if necessary. (45)
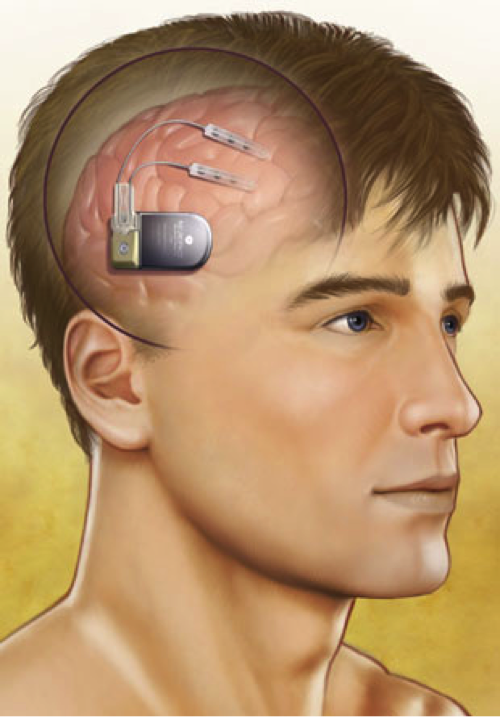 Image Courtesy NeuroPace, Inc. Caption: Illustration of a closed-loop, responsive neurostimulation system.
Being Evaluated for a Closed-Loop Stimulation System
Closed-loop stimulation may be offered to patients 18 years or older who have medically refractory epilepsy and are not candidates for traditional surgery. (40) It also may be offered to patients who have previously undergone traditional epilepsy surgery and continue to suffer from intractable epilepsy. (38, 36) Similarly, patients who had a VNS implanted may also be offered this treatment. (38, 47) For patients to be eligible, the exact location of their particular seizure focus must be known in order to direct stimulation there. (40) A closed-loop system is generally not recommended for epilepsy patients who have more than two distinct seizure foci. While a maximum of four electrodes can be implanted, only two can be connected to the device. (3)
Implantation of a Closed-Loop Stimulation System
Electrodes are only placed in brain areas shown to generate seizures, with the exact placement tailored to each patient. The main component, containing the battery and computer, is designed to be implanted within the skull itself. There are lower rates of infection and hardware failure from this placement compared to traditional systems (like VNS and DBS), in which the battery is implanted under the skin, but over the ribs in the chest. (49)
Implantation of the RNS® closed-loop system involves shaving the hair off one side of the head prior to making a fairly large incision in the scalp. Once the skull is exposed, two small holes (about 14mm in diameter) are made for the two electrodes. After the electrodes are in the proper position, covers are placed over the holes to help hold the electrodes in place. The location for implantation of the main component is then determined based on these two holes. A cavity for the main component is drilled out so that the device sits flush with the surface of the skull. The electrodes are connected to the main component and the incision is closed. (3)
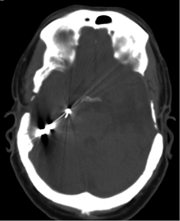 Caption: CT scan of a closed-loop, responsive neurostimulation system.
Risks Involved
During the RNS® System Pivotal Clinical Investigation, the rate of serious complications within the first month of implantation was reported to be 12%, which is comparable to the 15% risk of similar events in patients undergoing invasive monitoring with intracranial electrodes. (40) Common side effects include implant site pain and headache. Less commonly, patients will experience infection, bleeding, or death. (40) Although the complication rate increases to 18.3% over the course of the first 3 months after implantation, this complication rate is favorable in comparison to the overall rate of 36% associated with DBS for Parkinson’s disease or essential tremor. (50-54)
At this time, long-term experience beyond five years is currently very limited and the side effects of intermittent, long-term (also termed “chronic”) stimulation remain unclear.
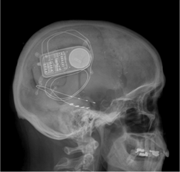 Caption: X-ray of a closed-loop, responsive neurostimulation system.
Expected Outcomes of Epilepsy Treatment with Deep Brain Stimulation (DBS)
There are currently three targets for DBS in epilepsy. These include the anterior thalamus (ANT), centromedian thalamus (CMT), and hippocampus (HIP). The ANT area has been the most widely studied and has been approved as a therapeutic target by the FDA. The CMT location, meanwhile, has promising data for severe generalized epilepsy. Finally, HIP is a target only suitable for patients with presumed onset from one or both mesial temporal areas of the brain. While this last approach is similar to RNS, the stimulation paradigm is different and their relative efficacy has not been directly compared.
For the ANT nucleus, there is Level 1 evidence from a two-year, randomized controlled trial, SANTE, which showed 54% of patients had at least 50% reduction in seizures, and 17% were seizure free at six months. (9) In a long-term follow up of five years, the SANTE study reported a 69% reduction in seizure frequency and 34% reduction in drug adverse events. (10) There was a 68% response (50% seizure reduction) at five years, versus 43% at one year. Also, by five years, 16% of the patients were seizure free for at least six months.
The CMT area has been investigated in the setting of Lennox Gastaut syndrome with severe generalized epilepsy, in which 13 patients were implanted with CMT DBS. (11) The study reported an 80% seizure reduction overall, with 10 of 13 patients having a 70% or more reduction at 18 months. This appears to be a good treatment modality in this group and seems to prevent drop attacks which often lead to injury if untreated.
Similar to RNS, DBS of the hippocampus has been shown to be efficacious for epilepsy. In a study population of nine temporal lobe epilepsy patients, seven had improved seizure frequency with high frequency stimulation after a mean follow up of 30.1 months, with a reduction of 66-97%. (12) Furthermore, all patients with mesial temporal sclerosis responded to hippocampal DBS. Future studies are needed to compare the efficacy to RNS and delineate which patients are more appropriate for respective treatments.
The DBS Device
The DBS device approved for ANT epilepsy applications is manufactured by Medtronic (67). The device includes a combination of two leads (one for each side, targeting the right and left nuclei), a pulse generator that produces the current to stimulate the area, and a connector that connects these leads from the head to the neck. There are a variety of leads and pulse generators available, including the Percept device (68), which is able to both stimulate and record signals adjacent to the stimulation area. While this is not a true closed-loop device like RNS, signal recordings allow for the possibility to adjust stimulation parameters based on analytics from recordings.
Other DBS systems used for DBS in Parkinson’s disease by 2021 included the Abbott and Boston Scientific systems (69). While those systems were not explicitly FDA-approved for an epilepsy application, they offer other technological features such as directional leads and remote programing that may lead to improved outcomes in select scenarios.
Being Evaluated for a DBS System
Epilepsy patients should undergo evaluation with a qualified neurologist including documentation of seizure type, frequency, and symptoms prior to starting medications. Patients who have epilepsy that is not responsive to medications are suitable candidates for consideration of surgical intervention. Studies have shown that failure of two antiepileptic drugs makes a trial of further pharmacologic therapy unlikely to succeed. (13) The process of evaluation for DBS, like any surgical procedure for epilepsy, begins with investigation of the root cause of symptoms. Fundamentally this involves imaging, neuropsychiatric evaluation, noninvasive monitoring, and in some cases invasive monitoring.
Imaging is one of the necessary first steps in evaluating the pathology driving seizures. Structural changes in the brain may be detected by MRI. These include mesial temporal sclerosis, low grade tumors, dysplasia, heterotopia, and cavernous malformations to name a few. It is important to determine if these are present, as they may suggest other treatment approaches more specific than DBS. In general, resection or ablation of a visible pathology that correlates with seizures leads to better outcomes than neurostimulation. To aid detection of seizure foci, PET/SPECT scans may help localize and lateralize seizures based on brain metabolism between or during seizure states respectively. Similarly, functional MRI (fMRI) may also help determine location of language function as well as help localize presumed epileptic regions based on changes in location of normal brain activity.
Similar to fMRI, neuropsychiatric evaluation can help determine memory, verbal, and special abilities that are affected by seizures. The dysfunctional area will often show deficit on neuropsychological testing.
Finally, EEG is vital to understanding seizure onset and spread. As a first step, patients should be evaluated in a long-term noninvasive EEG setting. This allows neurologist to review changes in bran rhythms before, during and after seizures, and relate these findings to videos of seizure symptoms. Often, between the imaging, clinical manifestation of the seizure, neuropsychological correlates and noninvasive recordings it is possible to reliably determine the location of seizure onset. However, sometimes further EEG recordings are necessary. This involves surgical implantation of subdural grids or stereotactic EEG leads (sEEG). Today, sEEG is favored due to similar results and a favorable complication profile. (14, 15)
If a seizure focus is found, it is preferable to remove the offending area through open surgery or ablation. In cases where resection is not possible, or there is no target for specific surgical evaluation, neurostimulation becomes the primary option for treatment. For patients with known onset areas, such as in the bilateral temporal lobes, RNS is a good option since detection and stimulation from these regions is feasible. Hippocampal DBS can also be used as an alternative in such scenarios when RNS is not available. When no target is known, DBS provides an excellent option to interrupt large networks of seizure spread.
Future Directions
A great deal of research remains to be done in closed-loop stimulation for the treatment of epilepsy. Both parts of the closed-loop – seizure detection and stimulation for halting seizures – must not only be individually optimized, but also work in unison with each other.
Seizure Detection versus Seizure Prediction The earlier stimulation is applied, the more effective it is at stopping abnormal EEG activity. (55) Therefore, both scientists and engineers are working to improve seizure detection, and possibly even predict them. The International Workshop On Seizure Prediction has been held since 2002 to gather and develop new methods. (11, 56)
Current algorithms analyze the EEG signal itself to detect changes that may represent seizure activity. While these systems are very sensitive to abnormalities in the EEG, they will often flag events that are not truly seizures. Therefore, extensive research is being done on signal processing that will help detect reliable changes in EEG signals that may not be otherwise be visible to the naked eye.
The promise of predicting seizures has been supported evidence of changes in EEG activity that occur 7-10 seconds before clinical symptoms. (11) Some researchers have even reported noticing changes in neuronal activity as early as 7 hours prior to the seizure itself. (15)
Optimization of Stimulation Parameters Stimulation parameters to halt seizure activity must also be improved. Currently, these parameters are based mainly on experience with DBS and with stimulation performed as part of an evaluation for surgical removal of a seizure focus.
Use as a Long-Term Monitoring Device As long as the patient continues to download data from the device onto the provided computer, the NeuroPace system has the ability to store EEG data over months and years. This type of long-term monitoring has already proven to be invaluable in our understanding of epilepsy. One study showed that on average it could take over a month before the complete spectrum of a patient’s seizure activity was seen. (61) Another demonstrated differences in the time of day that seizures from different parts of the brain occur. (64) A third study has also highlighted the negative effects of caffeine intake on EEG activity. (65) Overall, chronic monitoring with this device provides data in a naturalistic setting, which may be used to help epilepsy treatment teams with treatment decisions.
Technological Advances
The rise of new technologies will only improve our ability to create a more effective closed-loop neurostimulator. Improved wireless transmission of high quality data would significantly reduce the amount of hardware that needs to be implanted. This innovation would allow future versions of such neurostimulators to keep the larger elements of the device in a module to wear on a belt or a vest. (57)
Future applications of closed-loop systems may also be able to track the levels of certain chemicals in the brain. This information could be used independently or along with EEG data in predicting brain states consistent with activity prior to or during a seizure. (57)
The examples above are only a sample of the possibilities that exist for closed-loop neurostimulator systems. In all aspects of neuromodulation, continued collaboration between engineers and doctors will help to advance the field – and ultimately provide higher levels of care for patients.
Please note: This information should not be used as a substitute for medical treatment and advice. Always consult a medical professional about any health-related questions or concerns.
Resource
WIKISTIM – This free-to-use collaborative, searchable wiki of published primary neuromodulation therapy research was created in 2013 as a resource for the global neuromodulation community to extend the utility of published clinical research. The goals of WIKISTIM are to improve patient care and the quality of research reports, foster education and communication, reveal research needs, and support the practice of evidence–based medicine.
References
1. Choi H, Sell RL, Lenert L, et al. Epilepsy surgery for pharmacoresistant temporal lobe epilepsy: a decision analysis. JAMA : the journal of the American Medical Association. 2008;300(21):2497–505. 2. Cooke PM, Snider RS. Some cerebellar influences on electrically-induced cerebral seizures. Epilepsia. 1955;4:19–28. 3. Wu, C. and A.D. Sharan, Neurostimulation for the treatment of epilepsy: a review of current surgical interventions. Neuromodulation, 2013. 16(1): p. 10-24; discussion 24. 4. Vonck K, Boon P, Achten E, et al. Long-term amygdalohippocampal stimulation for refractory temporal lobe epilepsy. Annals of neurology. 2002;52(5):556–65. 5. Goetz, C.G., The history of Parkinson's disease: early clinical descriptions and neurological therapies. Cold Spring Harb Perspect Med, 2011. 1(1): p. a008862. 6. Benabid, A.L., et al., Long-term suppression of tremor by chronic stimulation of the ventral intermediate thalamic nucleus. Lancet, 1991. 337(8738): p. 403-6. 7. DeGiorgio, C.M., et al., Prospective long-term study of vagus nerve stimulation for the treatment of refractory seizures. Epilepsia, 2000. 41(9): p. 1195-200. 8. Wong, S., R. Mani, and S. Danish, Comparison and Selection of Current Implantable Anti-Epileptic Devices. Neurotherapeutics, 2019. 16(2): p. 369-380. 9. Fisher, R., et al., Electrical stimulation of the anterior nucleus of thalamus for treatment of refractory epilepsy. Epilepsia, 2010. 51(5): p. 899-908. 10. Salanova, V., et al., Long-term efficacy and safety of thalamic stimulation for drug-resistant partial epilepsy. Neurology, 2015. 84(10): p. 1017-25. 11. Osorio, I., et al., Subcortical (thalamic) automated seizure detection: A new option for contingent therapy delivery. Epilepsia, 2015. 56(10): p. e156-60. 12. Cukiert, A., et al., Seizure outcome after hippocampal deep brain stimulation in a prospective cohort of patients with refractory temporal lobe epilepsy. Seizure, 2014. 23(1): p. 6-9. 13. Chen, Z., et al., Treatment Outcomes in Patients With Newly Diagnosed Epilepsy Treated With Established and New Antiepileptic Drugs: A 30-Year Longitudinal Cohort Study. JAMA Neurol, 2018. 75(3): p. 279-286. 14. Mullin, J.P., et al., Is SEEG safe? A systematic review and meta-analysis of stereo-electroencephalography-related complications. Epilepsia, 2016. 57(3): p. 386-401. 15. Arya, R., et al., Adverse events related to extraoperative invasive EEG monitoring with subdural grid electrodes: a systematic review and meta-analysis. Epilepsia, 2013. 54(5): p. 828-39. 16. Weaver, F.M., et al., Bilateral deep brain stimulation vs best medical therapy for patients with advanced Parkinson disease: a randomized controlled trial. JAMA, 2009. 301(1): p. 63-73. 17. Elger, G., et al., Vagus nerve stimulation is associated with mood improvements in epilepsy patients. Epilepsy Research, 2000. 42(2-3): p. 203-210. 18. Krishna, V., et al., Anterior Nucleus Deep Brain Stimulation for Refractory Epilepsy: Insights Into Patterns of Seizure Control and Efficacious Target. Neurosurgery, 2016. 78(6): p. 802-11. 19. Warren, A.E.L., et al., Targeting the centromedian thalamic nucleus for deep brain stimulation. J Neurol Neurosurg Psychiatry, 2020. 91(4): p. 339-349. 20. FDA Okays VNS Therapy for Epilepsy in Children as Young as 4 Years. Medscape. https://www.medscape.com/viewarticle/882346. Published June 30, 2017. Accessed April 30, 2021.
22. Health C for D and R. Recently-Approved Devices - VNS Therapy System - P970003s050. 23. Good LB, Sabesan S, Marsh ST, et al. Control of synchronization of brain dynamics leads to control of epileptic seizures in rodents. International journal of neural systems. 2009;19(3):173–96. 24. Kossoff EH, Ritzl EK, Politsky JM, et al. Effect of an external responsive neurostimulator on seizures and electrographic discharges during subdural electrode monitoring. Epilepsia. 2004;45(12):1560–7. 25. Lesser RP, Kim SH, Beyderman L, et al. Brief bursts of pulse stimulation terminate after discharges caused by cortical stimulation. Neurology. 1999;53(9):2073–81. 26. Al-Otaibi FA, Hamani C, Lozano AM. Neuromodulation in epilepsy. Neurosurgery. 2011;69(4):957–79; discussion 979. 27. Davis R, Emmonds SE. Cerebellar stimulation for seizure control: 17-year study. Stereotactic and functional neurosurgery. 1992;58(1-4):200–8. 28. Litt B, Echauz J. Prediction of epileptic seizures. Lancet neurology. 2002;1(1):22–30. 29. Mormann F, Elger CE, Lehnertz K. Seizure anticipation: from algorithms to clinical practice. Current opinion in neurology. 2006;19(2):187–93. 30. Esteller R, Echauz J, Tcheng T, et al. Line length: an efficient feature for seizure onset detection. In: 2001 Conference Proceedings of the 23rd Annual International Conference of the IEEE Engineering in Medicine and Biology Society. Vol 2. IEEE; 2001:1707–1710. 31. D’Alessandro M, Esteller R, Vachtsevanos G, et al. Epileptic seizure prediction using hybrid feature selection over multiple intracranial EEG electrode contacts: a report of four patients. IEEE transactions on bio-medical engineering. 2003;50(5):603–15. 32. Litt B, Esteller R, Echauz J, et al. Epileptic seizures may begin hours in advance of clinical onset: a report of five patients. Neuron. 2001;30(1):51–64. 33. Echauz J, Padovani DA, Esteller R, et al. Median-based filtering methods for EEG seizure detection. In: Proceedings of the First Joint BMES/EMBS Conference. 1999 IEEE Engineering in Medicine and Biology 21st Annual Conference and the 1999 Annual Fall Meeting of the Biomedical Engineering Society (Cat. No.99CH37015).Vol 1. IEEE; 1999:439. 34. Gotman J. Automatic seizure detection: improvements and evaluation. Electroencephalography and clinical neurophysiology. 1990;76(4):317–24. 35. Viglione SS, Walsh GO. Proceedings: Epileptic seizure prediction. Electroencephalography and clinical neurophysiology. 1975;39(4):435–6. 36. Osorio I, Frei MG, Wilkinson SB. Real-time automated detection and quantitative analysis of seizures and short-term prediction of clinical onset. Epilepsia. 1998;39(6):615–27. 37. Osorio I, Frei MG, Sunderam S, et al. Automated seizure abatement in humans using electrical stimulation. Annals of neurology. 2005;57(2):258–68. 38. Anderson WS, Kossoff EH, Bergey GK, et al. Implantation of a responsive neurostimulator device in patients with refractory epilepsy. Neurosurgical focus. 2008;25(3):E12. 39. Gigante PR, Goodman RR. Alternative surgical approaches in epilepsy. Current neurology and neuroscience reports. 2011;11(4):404–8. 40. Morrell MJ. Responsive cortical stimulation for the treatment of medically intractable partial epilepsy. Neurology. 2011;77(13):1295–304. 41. Anon. Pivotal Trial Data Demonstrate NeuroPace RNS® System Reduced Seizures in People with Epilepsy. NeuroPace: News. 42. Sun FT, Morrell MJ, Wharen RE. Responsive cortical stimulation for the treatment of epilepsy. Neurotherapeutics: the journal of the American Society for Experimental NeuroTherapeutics. 2008;5(1):68–74. 43. Morrell M. Brain stimulation for epilepsy: can scheduled or responsive neurostimulation stop seizures? Current opinion in neurology. 2006;19(2):164–8. 44. Springer US, Bowers D, Goodman WK, et al. Long-term habituation of the smile response with deep brain stimulation. Neurocase. 2006;12(3):191–6. 45. Jobst BC. Electrical stimulation in epilepsy: vagus nerve and brain stimulation. Current treatment options in neurology. 2010;12(5):443–53. 46. Smith JR, Fountas KN, Murro AM, et al. Closed-loop stimulation in the control of focal epilepsy of insular origin. Stereotactic and functional neurosurgery. 2010;88(5):281–7. 47. Zhong X-L, Yu J-T, Zhang Q, et al. Deep brain stimulation for epilepsy in clinical practice and in animal models. Brain research bulletin. 2011;85(3-4):81–8. 48. Anon. NeuroPace submits PMA application for FDA approval of novel investigational device for epilepsy. NeuroPace: News.. 49. Andrews RJ. Neuromodulation: advances in the next five years. Annals of the New York Academy of Sciences. 2010;1199:204–11. 50. Hariz MI. Complications of deep brain stimulation surgery. Movement disorders: official journal of the Movement Disorder Society. 2002;17 Suppl 3:S162–6. 51. Joint C, Nandi D, Parkin S, et al. Hardware-related problems of deep brain stimulation. Movement disorders: official journal of the Movement Disorder Society. 2002;17 Suppl 3:S175–80. 52. Beric A, Kelly P, Rezai A, et al. Complications of deep brain stimulation surgery. Stereotactic and functional neurosurgery. 2001;77(1-4):73–8. 53. Oh MY, Abosch A, Kim SH, et al. Long-term hardware-related complications of deep brain stimulation. Neurosurgery. 2002;50(6):1268–74; discussion 1274–6. 54. Koller WC, Lyons KE, Wilkinson SB, et al. Long-term safety and efficacy of unilateral deep brain stimulation of the thalamus in essential tremor. Movement disorders: official journal of the Movement Disorder Society. 2001;16(3):464–8. 55. Motamedi GK, Lesser RP, Miglioretti DL, et al. Optimizing parameters for terminating cortical after discharges with pulse stimulation. Epilepsia. 2002;43(8):836–46. 56. Lehnertz K, Litt B. The First International Collaborative Workshop on Seizure Prediction: summary and data description. Clinical neurophysiology: official journal of the International Federation of Clinical Neurophysiology. 2005;116(3):493–505. 57. Shah RS, Chang S-Y, Min H-K, et al. Deep brain stimulation: technology at the cutting edge. Journal of clinical neurology (Seoul, Korea). 2010;6(4):167–82. 58. Zesiewicz TA, Sullivan KL, Arnulf I, et al. Practice Parameter: treatment of nonmotor symptoms of Parkinson disease: report of the Quality Standards Subcommittee of the American Academy of Neurology. Neurology. 2010 Mar 16;74(11):924-31. 59. Geller EB, Skarpaas TL, Gross RE, et al. Brain-responsive neurostimulation in patients with medically intractable mesial temporal lobe epilepsy. Epilepsia. 2017 Jun;58(6):994-1004. 60. Meador KJ, Kapur R, Loring DW, et al. RNS® System Pivotal Trial Investigators. Quality of life and mood in patients with medically intractable epilepsy treated with targeted responsive neurostimulation. Epilepsy Behav. 2015 Apr;45:242-7. 61. King-Stephens D, Mirro E, Weber PB, et al. Lateralization of mesial temporal lobe epilepsy with chronic ambulatory electrocorticography. Epilepsia. 2015 Jun;56(6):959-67. 62. Loring DW, Kapur R, Meador KJ, et al. Differential neuropsychological outcomes following targeted responsive neurostimulation for partial-onset epilepsy. Epilepsia. 2015 Nov;56(11):1836-44. 63. Spencer D. Responsive Neurostimulation and Cognition. Epilepsy Curr. 2016 Mar-Apr;16(2):98-100. 64. Spencer DC, Sun FT, Brown SN, et al. Circadian and ultradian patterns of epileptiform discharges differ by seizure-onset location during long-term ambulatory intracranial monitoring. Epilepsia. 2016 Sep;57(9):1495-502. 65. Mackow MJ, Krishnan B, Bingaman WE, et al. Increased caffeine intake leads to worsening of electrocorticographic epileptiform discharges as recorded with a responsive neurostimulation device. Clin Neurophysiol. 2016 Jun;127(6):2341-2. 66. Medtronic DBS System for Epilepsy - P960009/S219. FDA website. https://www.fda.gov/medical-devices/recently-approved-devices/medtronic-dbs-system-epilepsy-p960009s219. Updated May 3, 2018. Accessed April 19, 2021. 67. FDA Approves First-Of-Its-Kind Percept™ PC Neurostimulator with BrainSense™ Technology June 25, 2020. Medtronic website. https://newsroom.medtronic.com/news-releases/news-release-details/fda-approves-first-its-kind-percepttm-pc-neurostimulator. Published June 25, 2020. Accessed April 19, 2021. 68. Currently Available Deep Brain Stimulation Devices. Michael J. Fox website. https://www.michaeljfox.org/news/currently-available-deep-brain-stimulation-devices. Accessed April 19, 2021
April 21, 2021 https://www.neuromodulation.com/for-patients
|